Imaging innovation sharpens the view for radiotherapy clinics
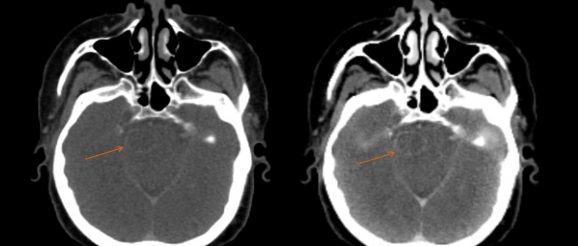
Rapid advances in imaging techniques based on computed tomography (CT) have underpinned a revolution in treating cancer patients using radiotherapy. An X-ray scan lasting just a few seconds yields an accurate 3D visualization of the patient’s internal anatomy, and delivers the critical information needed by medical physicists to calculate the optimal dose distribution for treating the tumour. Ongoing advances in CT technologies have enabled clinical teams to access higher quality images that have enabled more precise targeting of the tumour while minimizing the damage to healthy organs and tissues.
However, conventional CT images can sometimes lack the contrast needed to clearly distinguish between different types of soft tissue. That makes it difficult for radiation oncologists to precisely define the size and shape of the tumour, and to contour the nearby organs, tissues and blood vessels that need to be protected from ionizing radiation. Another notable limitation of the standard CT scans conventionally used in radiotherapy departments is that they only provide anatomical information, and so are unable to reveal functional processes that might provide some additional insight for treatment planning.
For cases that require enhanced soft-tissue contrast or functional information, CT is often combined with other imaging modalities such as magnetic resonance imaging (MRI) or positron emission tomography (PET). While Siemens Healthineers offers MRI and PET solutions that have been optimized for radiotherapy and for imaging patients in the intended treatment position, in some situations such complementary imaging methods may not be available. For making clinical decisions, particularly when critical organs are at risk – such as in the head-and-neck region, chest or the abdomen – improved soft-tissue contrast is crucial.
Now, however, a novel method that can improve the quality of CT images is starting to make a difference in radiotherapy clinics. Called dual-energy CT, or DECT, the technique acquires CT images from two different X-ray spectra rather than one. Conventional CT captures images using a single X-ray beam, which has a spectrum of photon energies with an average of around 70 keV and a typical peak energy of 120 keV. The image contrast of each material depends on how well it attenuates X-rays, which in turn depends on energy.
“At standard CT energies, most of the soft tissue we’re trying to image has very similar attenuation coefficients,” explains Jainil Shah, a research professional and R&D collaboration scientist at Siemens Healthineers. “That means that most of the organs look very similar when they are visualized on a CT image.”
Routes to dual-energy CT in radiotherapy
DECT mitigates this problem by generating images from X-ray scans taken over two different energy ranges. Since the first experiments that showed the potential of dual-energy CT in the 1970s, several different approaches have emerged for acquiring DECT images – and each one has its advantages and disadvantages. The simplest way is to scan the patient twice at two different energies, a technique known as “Dual Spiral” or “Twin Spiral”. Such consecutive scanning can offer excellent image contrast, since it allows for a wide separation between the two spectra. Because any movements of the patient between the two scans can introduce errors, a non-rigid image registration between the two images is performed automatically during post-processing to account for and compensate for any changes in position. “That makes Dual Spiral DECT most suitable for non-moving regions such as the brain and the head and-neck,” comments Shah.
Other techniques capture the two spectra simultaneously, recording all the information in a single scan and limiting the patient’s exposure to X-ray radiation. One option offered by Siemens Healthineers is to split the X-ray beam using a filter in the scan direction, creating two separate beams with different average energies. Such TwinBeam technology offers a wide field-of-view, but the use of a filter limits the spectral separation and therefore the image contrast that can be achieved.
The third option available from Siemens Healthineers is a CT scanner that exploits two X-ray sources operating at different energies, each one coupled to its own detector. This Dual Source approach offers better spectral separation than TwinBeam technology, and therefore sharper images for treatment planning, as well as more X-ray power in each of the separate beams. The field-of-view is slightly smaller because the equipment needs to accommodate two separate X-ray tubes, which needs to be considered when imaging larger regions of the body.
These DECT systems are already routinely used in radiology clinics for diagnostic imaging, while ongoing improvements to the scanners and the software have made it much easier for radiotherapy centres to integrate the technique into their clinical workflow. “The scan can be acquired by any technician in the clinic, and all the information needed by the radiation oncologist is generated automatically,” explains Shah. “Clinical workflows can be set up in the software to automatically perform additional post-processing and image reconstruction from a single scan.”
Capturing X-ray spectra with two different energy distributions makes it possible to reconstruct an image at any single energy. This yields a series of virtual monoenergetic images (VMIs), also called Monoenergetic Plus – at energies ranging from around 40 keV up to 190 keV – that can be used to optimize the soft-tissue contrast. “The energy can be easily changed with a slider bar in the software,” explains Shah. “The radiation oncologist can decide which energy provides the best contrast for contouring organs.”
Shah says that dual-energy CT is also able to provide some functional information about dynamic processes inside the body, such as perfusion within the lungs or the uptake of iodine in different organs and blood vessels. As an example, capturing X-ray spectra over two different energy ranges makes it possible to determine the material composition, since the attenuation of X-rays in each material is dependent on energy.
“That means you can do things like remove bone from the image, or distinguish between fat and liver tissue,” says Shah. “From the material composition you can predict the electron density of the material (Rho image), which is the key information that is used for dose calculations in radiation therapy.” For proton therapy, meanwhile, Siemens Healthineers offers a specific reconstruction called “DirectSPR” that calculates the stopping-power ratio from dual-energy CT.
Into the clinic
Ongoing advances in technology and software are now enabling medical teams to integrate dual-energy CT into their clinical practice.
Beth Bradshaw Ghavidel, Emory University, USA – DECT for head-and-neck patients
Beth Bradshaw Ghavidel, one of the lead medical physicists at Emory University, says that TwinBeam DECT is primarily used for head-and-neck patients, in which VMIs at higher energies can help to remove the artefacts that arise from metal objects inside the body, such as dental fillings (DECT is compatible with iterative metal artefact reconstruction, iMAR). “It is easy to set up the desired dual-energy CT workflows on the scanner and allow automatic post processing,” says Bradshaw Ghavidel. “Depending on what CT scans are needed, the dosimetrist can select specific studies for import. At this time, we have not needed to alter our clinical workflow for additional imaging studies.”
Lili Chen, Fox Chase Cancer Center, USA – DECT for intracranial target delineation and rectal tumours
Lili Chen at Fox Chase Cancer Center has also been exploring the potential of dual-energy CT to boost the image quality for different disease sites. When imaging the head and neck, she found – like Bradshaw Ghavidel – that a VMI at 190 keV offers an effective way to reduce the artefacts caused by dental fillings and iodine uptake in the soft palate. Chen has also compared DECT images of brain tumours in 34 different patients with those taken with MRI. When an iodine contrast agent was used, she found that a VMI at 40 keV can reveal metastases in the brain that are not detected by conventional CT, or by VMIs at higher energies. What’s more, in this research study the brain tumour volume derived from the 40 keV DECT image was comparable to that obtained from MRI.
“Meaningful clinical differences were found on CT images with contrast, with the 40 keV image delineating the tumour much more clearly than images taken at other energies,” Chen comments. “Our results suggest that dual-energy CT with contrast may be used for intracranial target delineation in radiotherapy treatment planning.” Analysis of a rectal tumour also revealed obvious differences between images taken at different energies, with the one taken at 40 keV clearly showing the necrotic area of the tumour and indicating that cancerous cells had spread to the adjacent seminal vessels.
Xiaofeng Yang, Emory University Hospital – DECT image contrast for contouring head-and-neck cases
Also at Emory University, Xiaofeng Yang has been working with Bradshaw Ghavidel and other colleagues to investigate whether dual-energy CT can improve the precision of a deep-learning model they have developed for automatic organ segmentation. In a recent research study, they designed a neural network that exploits DECT data to automatically segment 19 organs-at-risk in the head and neck, and trained the model using manual contours produced for 66 patients with carcinomas in different disease sites. Automatic contours generated with the DECT-based model were then compared to manual contours produced by a physician, as well as to those obtained using the same model developed at Emory University Hospital with conventional CT data. “DECT-based segmentation of organs-at-risk has the potential to facilitate the current head-and-neck cancer radiation therapy workflow in treatment planning,” concludes Yang.
George Noid, Medical College Wisconsin (MCW), USA – DECT in clinical routine and for tracking the effect of treatment
George Noid, a medical physicist at MCW, says that dual-energy CT is now in routine clinical use for almost all cancer patients, particularly those needing treatment in the abdomen or chest. “We use the VMI reconstructions to enhance the image contrast,” he says. “As well as abdominal and thoracic patients, we’ve found it really useful for pre-operative breast cancer and other rare disease sites in the abdomen, such as the adrenal glands.”
One particularly gnarly problem that Noid hopes to address in the future is to improve the images used to plan the treatment of pancreatic cancer. “We want to deliver as much radiation as possible to the pancreatic head, but the major limiting factor is the amount of radiation that we can deliver to the adjacent duodenum,” he explains. “That makes it clinically important to accurately define the edge between the pancreatic head and the duodenum.” In a recent research study, Noid and colleagues compared conventional CT data with dual-energy CT scans of 10 patients being treated for pancreatic cancer, and in each case the image contrast was enhanced by injecting iodine-based contrast media into the patient before the scan. They found that the image contrast was boosted by a factor of 2.8 for the VMI at the lowest possible energy of 40 keV, while another important indicator of image quality, the contrast-to-noise ratio, was also maximized at this energy. Images from other treatment sites, including the liver, breast and thymus, also showed that the tumours were more clearly visible at 40 keV than at higher energies.
Noid is also investigating whether quantitative data extracted from dual-energy CT images could be used as an indicator of how well a patient is responding to treatment. “It has been shown that the aggressiveness of pancreatic cancer is correlated to the extracellular volume (ECV) fraction, which can be calculated from a DECT scan,” he explains. In a recent research study, which won the Best in Physics award at AAPM 2021, Noid and colleagues used DECT images acquired at weekly treatment sessions to calculate the ECV fraction. For 12 pancreatic cancer patients, the study revealed a correlation between the ECV fraction and the concentration of a cancer antigen found in the blood, suggesting that regular DECT scanning could be used to track the effect of treatment. “That offers the potential of stratifying your patient’s risks based on calculations of the ECV fraction,” Noid explains. “That information could help to drive your clinical decisions, such as giving a higher dose to a patient who has a more aggressive disease. We are not yet using that in our clinical workflow, but that’s the idea.”
Noid is confident that DECT has the potential to deliver more quantitative data that in the future could be used to analyse the properties of the tumour. “Unlocking that information would be clinically very useful,” he says. “We’re starting to see that DECT has the power to access that functional information, and I think there’s much more we can do.”
Note that the results by Siemens Healthineers’ customers described herein are based on results that were achieved in the customers’ unique setting. Since there is no “typical” hospital and many variables exist (eg. hospital size, case mix, level of IT adoption) there can be no guarantee that other customers will achieve the same results.
The post Imaging innovation sharpens the view for radiotherapy clinics appeared first on Physics World.