A bacterial enzyme converts carbon dioxide into carbon compounds 20 times faster than plant enzymes do during photosynthesis – Innovation Toronto
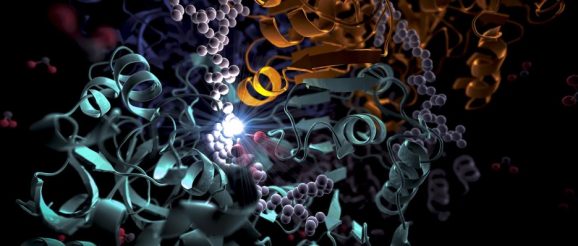
Portraits of an enzyme
Wakatsuki and his group had been investigating a related system, nitrogen fixation, which converts nitrogen gas from the atmosphere into compounds that living things need. Intrigued by the question of why ECR enzymes were so fast, he started collaborating with Erb’s group to find answers.
Hasan DeMirci, a research associate in Wakatsuki’s group who is now an assistant professor at Koc University and investigator with the Stanford PULSE Institute, led the effort at SLAC with help from half a dozen SLAC summer interns he supervised. “We train six or seven of them every year, and they were fearless,” he said. “They came with open minds, ready to learn, and they did amazing things.”
The SLAC team made samples of the ECR enzyme and crystallized them for examination with X-rays at the Advanced Photon Source at DOE’s Argonne National Laboratory. The X-rays revealed the molecular structure of the enzyme – the arrangement of its atomic scaffolding – both on its own and when attached to a small helper molecule that facilitates its work.
Further X-ray studies at SLAC’s Stanford Synchrotron Radiation Lightsource (SSRL) showed how the enzyme’s structure shifted when it attached to a substrate, a kind of molecular workbench that assembles ingredients for the carbon fixing reaction and spurs the reaction along.
Finally, a team of researchers from SLAC’s Linac Coherent Light Source (LCLS) carried out more detailed studies of the enzyme and its substrate at Japan’s SACLA X-ray free-electron laser. The choice of an X-ray laser was important because it allowed them to study the enzyme’s behavior at room temperature – closer to its natural environment – with almost no radiation damage.
Meanwhile, Erb’s group in Germany and Associate Professor Esteban Vo?hringer-Martinez’s group at the University of Concepción in Chile carried out detailed biochemical studies and extensive dynamic simulations to make sense of the structural data collected by Wakatsuki and his team.
The simulations revealed that the opening and closing of the enzyme’s two parts don’t just involve molecular glue, but also twisting motions around the central axis of each enzyme pair, Wakatsuki said.
“This twist is almost like a rachet that can push a finished product out or pull a new set of ingredients into the pocket where the reaction takes place,” he said. Together, the twisting and synchronization of the enzyme pairs allow them to fix carbon 100 times a second.
The ECR enzyme family also includes a more versatile branch that can interact with many different kinds of biomolecules to produce a variety of products. But since they aren’t held together by molecular glue, they can’t coordinate their movements and therefore operate much more slowly.
“If we can increase the rate of those sophisticated reactions to make new biomolecules,” Wakatsuki said, “that would be a significant jump in the field.”
From static shots to fluid movies
So far the experiments have produced static snapshots of the enzyme, the reaction ingredients and the final products in various configurations.
“Our dream experiment,” Wakatsuki said, “would be to combine all the ingredients as they flow into the path of the X-ray laser beam so we could watch the reaction take place in real time.”
The team actually tried that at SACLA, he said, but it didn’t work. “The CO2 molecules are really small, and they move so fast that it’s hard to catch the moment when they attach to the substrate,” he said. “Plus the X-ray laser beam is so strong that we couldn’t keep the ingredients in it long enough for the reaction to take place. When we pressed hard to do this, we managed to break the crystals.”
An upcoming high-energy upgrade to LCLS will likely solve that problem, he added, with pulses that arrive much more frequently – a million times per second – and can be individually adjusted to the ideal strength for each sample.
Wakatsuki said his team continues to collaborate with Erb’s group, and it’s working with the LCLS sample delivery group and with researchers at the SLAC-Stanford cryogenic electron microscopy (cryo-EM) facilities to find a way to make this approach work.