Spallation sources drive vacuum innovation
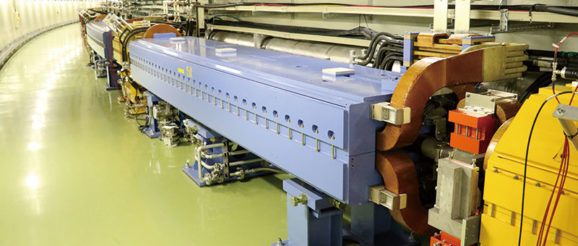
A new generation of neutron sources is providing scientists with unprecedented power to probe the structure and properties of materials ranging from biomolecules through to superconductors. Spallation sources recently built in the US, Japan and China are now the brightest neutron sources in the world, while the European Spallation Source (ESS) now being built in Lund, Sweden, will set the bar even higher when it comes online for users in 2023.
But these powerful new instruments present a challenge for the engineering systems needed to support them. High neutron fluxes generate large amounts of radiation, which creates issues for critical infrastructure such as the vacuum systems needed to maintain ultrahigh vacuum conditions within the experimental facility. “We need to build reliable vacuum equipment that won’t be damaged by radiation,” says Andreas Schopphoff of Pfeiffer Vacuum, who has worked closely with users to devise custom vacuum solutions for this new breed of spallation sources. “It can cause problems both for the electronic systems used to control vacuum pumps and gauges, and the materials we use to build them.”
Neutrons reveal material secrets
Neutrons are widely used to study material properties because they penetrate deep into the sample, scattering off atomic nuclei to generate data that researchers can analyse to determine the detailed structure within the material. Neutrons also have the right wavelength to transfer their momentum and energy to the particles inside the sample, which provides valuable information about fundamental vibrations that control chemical bonding and magnetic interactions. What’s more, neutrons have a nuclear spin that makes them very sensitive to the location and orientation of magnetic moments in materials.
While neutron sources have been available for many years, this new generation of spallation sources can deliver neutron beams that are orders of magnitude more powerful than previous designs based on nuclear reactors – which can speed up experiments and enable scientists to probe materials and their interactions with more detail than ever before.
The most intense reactor-based source available today, the Institut Laue-Langevin in Grenoble, France, achieves a power of 58 kV, while the ESS will reach 5000 kV by smashing a high-energy proton beam into a solid target made of tungsten. The force of the impact creates free or “spalled” neutrons, which are then slowed and collimated into an intense neutron beam that can easily be pulsed for neutron scattering experiments. Other spallation sources operate in a similar way, but can use different acceleration methods and different types of target – although heavy metals are chosen for their ability to absorb radioactive species produced during the spallation process.
But radiation is still a major concern for these high-power neutron sources. At the Japanese Proton Accelerator Research Complex (J-PARC) – the most intense neutron source in operation today – radiation levels can reach millions of grays in the vicinity of the neutron beam.
According to Schopphoff, such high levels of ionizing radiation creates two major problems for vacuum pumps and gauges stationed close to the neutron source. “First, we cannot connect electronic systems directly to vacuum pumps or gauges because the radiation would cause them to fail and stop working,” he says. “We also need to think about eliminating certain materials from the pump design, such as Teflon, that would also be affected by high levels of radiation.”
Finding radiation-resistant solutions
The solution for the electronics, says Schopphoff, is to position them a long way from the pumps. At the China Spallation Neutron Source, for example – which has just completed its first round of operations – some of the vacuum gauges were connected to their controllers via cables up to 220 m long. Vacuum generation is equally important, and both the turbopumps and the backing pumps must be designed to be radiation resistant.
“For some applications it’s important to bring enough electrical power to the pump,” he explains. “So we have to install large cables, particularly for the backing pumps. At J-PARC we worked with the project team to develop an electronic system specifically for their needs.”
Meanwhile, the effect of ionizing radiation on materials can demand a whole new approach to pump design. Most large-scale experimental facilities exploit high-speed turbopumps to maintain UHV conditions, but to be effective they need a backing pump to reduce atmospheric pressure to a “rough” vacuum. In the case of J-PARC, the project team were concerned that the Teflon used within the two most common types of backing pump – oil-sealed or dry backing pumps – would become more brittle when exposed to radiation.
The solution, says Schopphoff, was to introduce an oil-free backing pump design called the multistage roots pump, which reduces the air pressure via a series of rotors spinning at speeds of up to 6000 rpm. “A multistage roots pump does not have any kind of sealing material, so there is no need to use Teflon,” explains Schopphoff. “It is a combination of up to six stages of roots blowers, which can compress the gas from medium vacuum to atmospheric pressure.” The frictionless operation of the pump also prevents wear and reduces the need for maintenance, which is particularly useful when the pump does not include any Teflon.
According to Schopphoff, J-PARC was the first neutron facility to exploit a multistage roots pump for the initial stage of the pumpdown. “Since then we have developed a new model that is attracting more interest from other spallation sources, particularly the ESS, as well from other customers who need vacuum systems that are exposed to high levels of radiation.”
New challenges at the ESS
For the ESS, the higher neutron flux means that radiation will be an even greater problem. As with the backing pump, the ESS project team is also concerned with the tiny amounts of Teflon that are used inside the turbopumps. As an example, explains Schopphoff, a turbopump incorporates an electrical motor that is insulated with Teflon. Any damage to the insulation could cause an electrical short circuit that stops the pump from working and interrupts the experimental run.
“We are currently discussing the options with the ESS project team,” says Schopphoff. “It would be pretty much impossible to remove all the Teflon from a turbopump, but it might be possible in some places. We have to look at the amount of effort it would take to change all these materials, or whether it might be better to leave them in.”
One of the problems both for Pfeiffer Vacuum and the ESS project team is that there is no easy way to test the long-term behaviour of turbopumps when exposed to the radiation levels expected at the facility. “This will be the first time that a vacuum system will be used in these conditions, and so we have to rely on the users and their experience of what types of materials can be used,” says Schopphoff. ”That’s the reason we always need to have close communication with the users.”
The post Spallation sources drive vacuum innovation appeared first on Physics World.