YouTube Videos -> Why mice are the best candidates for research? @ Why do we use mice to study human diseases? @ Some Links, Images and Websites – Science, Technology and Innovation – Time – Data – World @ Rodrigo Nunes Cal
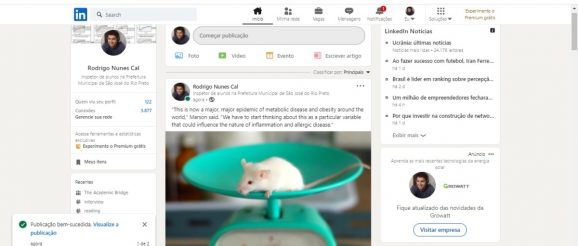
Obesity can turn a helpful immunotherapy into a harmful one, mouse study shows
By Jonathan Wosen March 30, 2022Reprints
Researchers have long known that obesity rewires the immune system. Now a new study suggests these effects can be so profound they could turn a drug meant to treat a common inflammatory disease into one that makes things worse.
Scientists discovered that mice with atopic dermatitis, a painful and itchy skin rash often triggered by an allergic reaction, were worse off if they were obese. A closer look at their immune responses led to a surprise finding: Different immune cell types drove the disease in obese versus lean mice. That caused a standard treatment to exacerbate symptoms in heavier animals, but adding another drug that made the immune response of the obese mice resemble those of lean animals made the treatment regimen work again.
The authors found some preliminary evidence that obesity alters immune responses to allergic diseases similarly in people, too. But it’ll take additional work to show whether the findings, published Wednesday in the journal Nature, hold up in humans.
These early-stage results aren’t cause for alarm among people concerned about whether their medications are working, cautions Alexander Marson, director of the Gladstone-UCSF Institute of Genomic Immunology, one of the study’s senior authors. But he added that the work raises the possibility that researchers may need to tailor treatments depending on a patient’s metabolism.UPCOMING EVENT
The STAT Madness crowd favorite
On April 20, hear researchers from the winning team discuss their work. Register Now!
“This is now a major, major epidemic of metabolic disease and obesity around the world,” Marson said. “We have to start thinking about this as a particular variable that could influence the nature of inflammation and allergic disease.”
More than 40% of U.S. adults have obesity, according to the Centers for Disease Control and Prevention, with some studies estimating that figure could jump to nearly 50% by 2030.
That’s likely to lead to a rise in all sorts of health conditions — and not just type 2 diabetes and heart disease. There’s growing evidence that obesity can worsen everything from asthma to various cancers to Covid-19. Part of the problem, researchers believe, is that obesity creates a low level of inflammation throughout the body.
To study the issue more closely, a team of researchers from the Salk Institute, Gladstone Institutes, and the University of California, San Francisco, zeroed in on atopic dermatitis. The inflammatory disease is the most common form of eczema and leads to dry and itchy patches of scaly skin across a person’s hands, feet, and eyelids, among other places.
Researchers triggered the disease in mice by injecting their ears with chemicals that sparked an inflammatory response in the skin. They found that disease was more severe in obese animals, whose ears swelled more than twice as much as lean mice.
Scientists then looked at exactly which immune cells were driving disease in both sets of mice. They discovered that obese mice didn’t simply have a higher level of inflammation — their disease was driven by a completely different set of cellular signals.
“That was the real surprise,” Marson said.
The unexpected finding came from looking at T helper cells. Like generals coordinating a military attack, they control the scale and strategy of an immune response. They do so by releasing chemicals known as cytokines, essentially marching orders for cells.
In lean mice, the authors found that disease was driven by a class of T helper cells known as Th2. That’s what they expected. But in obese mice, the response came from a distinct group known as Th17. Each subset of cells spits out different cytokines, and when scientists tried to treat obese mice with a pair of antibodies that blocks two key Th2 cytokines, they made things worse. The animals started to develop little pustules along their ears, a sign of severe disease.
Their next challenge? Understanding what happened, and whether they could reverse it. Researchers found that a protein called PPAR-gamma, which turns on key genes in Th2 cells, was less active in obese mice. So they treated these mice with rosiglitazone, a drug that revs up PPAR-gamma and is typically used to help people with type 2 diabetes respond to insulin, and the animals began responding to Th2-targeted treatment.
Turning an ineffective treatment back into a useful one was a key piece of this study, said Akiko Iwasaki, a Yale immunologist who wasn’t part of this effort. But she cautions that all the mice studied were male; in people, atopic dermatitis mainly affects women.
“It would have been good to see if there are any sex differences in the impact of obesity on atopic dermatitis disease and treatment,” said Iwasaki in an email to STAT.
The findings have potential real-world implications. Rosiglitazone has already been approved by the Food and Drug Administration and is sold under the name Avandia by U.K. pharma giant GlaxoSmithKline. And French drugmaker Sanofi markets Dupixent, a drug approved for eczema that blocks the same two Th2 cytokines Marson’s team went after in this study: IL-4 and IL-13.
Marson hasn’t spoken with any companies about the report, as there’s still plenty of follow-up work his team needs to do to see whether these results hold up in people. The authors looked back on previously collected data and found some signs of increased Th17 responses and decreased Th2 activity in obese patients with atopic dermatitis and asthma, respectively, but that evidence is preliminary.
He says a key next step would be to look at patients with many different types of allergic inflammation to see whether the immune signals driving disease are consistently different in people who have obesity. Marson adds that researchers could then sift through current data on how people respond to Th2-blocking treatments to see if the effectiveness of these drugs varies depending on a person’s body mass index. Those findings could potentially lead to future clinical trials that test the safety and effectiveness of therapies tweaked to better treat people with obesity.
“Do we have to think about different targets in the setting of altered metabolic states and obesity?” Marson said. “This hypothesis here is thought-provoking and changes the way that we see these things. But [it’s] not yet at a point where this could actually motivate specific treatment decisions.”
About the AuthorReprints
West Coast Biotech & Life Sciences Reporter
Jonathan Wosen is STAT’s West Coast Biotech & Life Sciences Reporter
Exclusive biopharma, health policy, and life science analysis. Start your 30-day free trial today.
Mo Med. 2013 May-Jun; 110(3): 207–211.
PMCID: PMC3987984
The Mighty Mouse: The Impact of Rodents on Advances in Biomedical Research
Mice and rats have long served as the preferred species for biomedical research animal models due to their anatomical, physiological, and genetic similarity to humans. Advantages of rodents include their small size, ease of maintenance, short life cycle, and abundant genetic resources. The Rat Resource and Research Center (RRRC) and the MU Mutant Mouse Regional Resource Center (MMRRC) serve as centralized repositories for the preservation and distribution of the ever increasing number of rodent models.
Perceptions of Mice and Rats: An Introduction
Mention mice and rats to most people and images of unsanitary conditions and urban decay come to mind. Rats have been vilified as the carriers of the infected fleas that led to the dreaded Black Plague that decimated Europe, North Africa and Central Asia in the fourteenth century. It has been suggested recently that an apology is in order and that other influences, not rodents, were to blame.1 More recently, infected mice have resulted in Hantavirus outbreaks including the recent scare in Yosemite National Park where many campers contracted the deadly virus from mice living in the cabins.2
For many people, exposure to rodents consists of seeing them used as food for snakes or birds of prey at the zoo, or having to deal with unwanted rodents invading their dwellings. A kinder image of rats and mice sometimes appears in literature – think of the rodents in E.B. White’s beloved books: the adventurous mouse Stuart in Stuart Little or the gluttonous rat, Templeton, of Charlotte’s Web fame. Recall the heroic cartoon character Mighty Mouse who always “saved the day” and of course, the best known mouse of all, Disney’s Mickey Mouse.
What is not always appreciated is the extraordinary impact that laboratory mice and rats have on biomedical research. They are often the preferred animal model for studies of human disease and the standard species of choice for pre-clinical trials.
Comparative Medicine Research
Comparative medicine is built on the ability to use information from one species to understand the same processes in other species. Basic biomedical research involves the characterization of genes/proteins, the study of anatomical and physiological functions and the characterization of normal and pathological states in a variety of animal species. This knowledge is then applied to understanding these same processes in humans. Likewise, information gained in the field of human medicine can be mined to advance veterinary medicine because of the commonalities among species that form the basis of comparative medicine.
Laboratory rats and mice provide ideal animal models for biomedical research and comparative medicine studies because they have many similarities to humans in terms of anatomy and physiology. Likewise, rats, mice, and humans each have approximately 30,000 genes of which approximately 95% are shared by all three species.3–6 The use of rodents for research purposes has economic advantages: mice and rats are relatively small and require little space or resources to maintain, have short gestation times but relatively large numbers of offspring, and have fairly rapid development to adulthood and relatively short life spans. For example, mice have a gestation period of approximately 19–21 days; can be weaned at three to four weeks of age, and reach sexual maturity by five to six weeks of age, allowing large numbers of mice to be generated for studies fairly quickly.
The use of rodents also provides advantages related to the wealth of genetic information available to scientists. The human genome was sequenced in 2001,4, 5 with those of the mouse and rat following in 2002 and 2004 respectively.3, 6 The availability of the complete nucleotide sequences for all three species has enabled genome-wide comparisons across species which have been critical for the identification and characterization of genes. The ability to use sophisticated molecular genetic techniques to manipulate the genes in mice and more recently rats, allows genes to be “knocked out” (no expression) or expressed at designated times of development or in select tissues in order to better understand their normal function and/or role in disease.
Mice as Models to Study Human Disease: Hereditary Deafness
The identification of genes responsible for hereditary deafness provides an excellent example of the utility of mice for studying human disorders. In humans, hearing loss is the most common sensory deficit, with congenital deafness occurring in one per every 1,200 to 2,000 live births. 7 Over a hundred different genes are involved in non-syndromic hearing loss.8 The mouse has been instrumental in identifying and characterizing a large number of these genes.
Traditionally, it has been difficult to study the genetics of hearing loss and deafness in humans due in part to the lack of large families or large numbers of affected individuals for studies, the issue of genetic heterogeneity (the phenomenon in which a single genetic disorder, in this case deafness, can be due to mutations or defects in a number of different genes), and the general inability to perform detailed anatomical analysis on human ears, particularly inner ears. Especially problematic is the difficulty in ascertaining what damage is due to inherited factors versus environmental factors such as exposure to loud noise or infections.
The mouse allows investigators to circumvent all of these problems. Genetically identical inbred strains of mice carrying a single mutated gene are particularly valuable in identifying gene function. Many mice carrying mutations related to hearing loss have been identified over the years because of the often obvious phenotype (physical characteristics) of a deaf mouse: failure to respond to noise as indicated by the lack of Preyer’s reflex (ear flick), unusual head tossing and circling (literally running around in a circle) behavior. This latter characteristic is the manifestation of the vestibular defects that are common in mouse deafness mutants. Unlike with humans, mice can be purposely bred to specific mates in order to generate many offspring with the desired genetic make-up (genotype). Because the mice are raised in controlled, pathogen-free environments, the effect of environmental factors can be highly controlled. Thus, we can attribute any hearing deficits completely to the genetic mutation. Because of the identical anatomical structures, findings in the mouse can be directly correlated to the expected pathology in humans. Lastly, using comparative genomic techniques, identification of a gene responsible for deafness in the mouse allows the equivalent (orthologous) gene to be identified in people. Beyond these studies to identify genes important in auditory development and function, the mouse strain can serve as a model to further explore the biological function of the gene and better understand its role in the auditory system. To date, over 55 non-syndromic human deafness genes have been identified9 and in every case, a corresponding mouse mutant serves as a model for that particular form of deafness (http://hearingimpairment.jax.org/models).
Advantages of Rats in Biomedical Research
Since mice are small in size and generally cost less to maintain, and because the tools to genetically manipulate their genomes have been available since the 1980s, mice are often the first choice as a rodent model. However, there are many areas of investigation where rats are preferred, including cardiovascular research, behavioral studies and toxicology.10
Cardiovascular disease is the leading cause of death and morbidity in developed countries (http://www.who.int/cardiovascular_diseases) and it is typically multifactorial (caused by combinations of genetic and environmental factors).11 Rats are often the preferred rodent model for cardiovascular research where their larger size is an advantage, especially for facilitating surgical procedures and other types of testing. Many unique strains of rats have been generated that model the complex nature of human obesity, diabetes, and cardiovascular disease and therefore in this case, rats provide excellent animal models for the study of these diseases.12,13
Rats are commonly used for behavioral studies because they are much more social than mice and their behavior better mimics behavior seen in humans. For example, expansion of a three-base pair sequence in the FMR1 gene is responsible for Fragile X syndrome, the most common cause of inherited intellectual disability in humans.14 This expansion leads to methylation of the FMR1 gene, essentially shutting it down such that the gene is not expressed.15 Defects in this gene are a known genetic cause of autism.16 When the FMR1 gene is knocked out in mice, they exhibit elevated social interactions.17 However when the same gene is knocked out in rats, they become less engaged in social play and emit fewer vocalizations during play sessions. These social impairments more closely parallel social behavior symptoms seen in humans with FMR1 mutations. Affected rats also display compulsive chewing behavior. Compulsive and repetitive behaviors are core symptoms in autism spectrum disorders (http://www.sageresearchmodels.com). Thus, in this case, the rat is the more appropriate rodent model.
Choosing the Best Species
In the past, the use of the mouse often eclipsed that of the rat because of the availability of better molecular techniques to manipulate the mouse genome. Recent advances in genetic tools to create knockout rat models promise to eliminate these barriers and may lead to an increase in the use of rats for a wider variety of biomedical research. Ultimately, the choice of rodent model depends on which species more closely recapitulates the symptoms and disease process seen in humans. It is clear that rats are not simply huge mice and that each species has advantages and disadvantages that often depend on the particular process or gene being studied. From a translational medicine standpoint, it is particularly critical to choose the appropriate model because a tremendous amount of money is spent testing drugs and therapies that ultimately fail at various stages of pre-clinical and clinical trials. One reason for this is that results obtained in animal trials do not always accurately reflect outcomes in humans.
It has been estimated that new drugs take an average of 15 years to go from discovery to market at an average cost of $900 million.18 Based on a report from the Tufts Center in 2001, it is estimated that of 5,000–10,000 compounds that enter the development pipeline, 250 will make it to preclinical trials and of those, only five will move into human clinical trials. Of those five, on average, only one will actually make it to market. The investment losses of money and time associated with the four failed drugs is huge. Interestingly, a retrospective study of several best selling drugs found that the mouse knock out phenotypes of the targets of these drugs correlated well with known drug efficacy. The therapeutic effect observed in the knock out model was often a good indicator of success in the clinic.19 This supports the notion that establishing a more specific and sensitive preclinical trial paradigm based on the best animal models will reduce drug development costs and more importantly, reduce the risk to human subjects in clinical trials.
Rodent Resource Centers
The use of rodents in biomedical research continues to rise and the number of unique strains and models is increasing tremendously as individual investigators and large federally funded multi-group projects generate increasing numbers of genetically engineered mice and rats.
The University of Missouri has the unique distinction of being the home of three National Institute of Health (NIH)-funded animal resource centers: The Rat Resource and Research Center (RRRC), the MU Mutant Mouse Regional Resource Center (MMRRC) and the National Swine Resource and Research Center (NSRRC). The RRRC and NSRRC are the only Centers of their kind in the United States. The MMRRC is part of a consortium of four regional centers located throughout the U.S. The purpose of the two rodent-centric Resource Centers is to serve as repositories for rat and mouse strains/stocks that are important for use in biomedical research. The Centers 1) import these important animal models, 2) cryopreserve embryos and/or sperm as a means to store and bank the models, and 3) serve as distribution centers to send the animals or cryopreserved materials to investigators worldwide who use these models in their research.
These repositories came into existence as a means to centralize the storage and distribution of important rodent animal models. The time and money used to create genetically modified animal models and characterize them is quite large; it is therefore important for them to be readily available to the scientific community for further research. The Resource Centers take the burden from the individual investigators of preserving the strains and shipping the animals to other researchers. Importantly, the Resource Centers use strict quality control measures to ensure that the genetics and clean health status of these models are monitored and maintained at the highest standards.
Currently, the most highly requested rats that are distributed by the RRRC are strains that contain a genetic modification consisting of the addition of a fluorescent reporter gene (enhanced green fluorescent protein or EGFP) within their genome. The consequence of this genetic addition is that every cell in these rats expresses the green fluorescent protein, allowing them to be readily observed by fluorescence microscopy (see Figure 1). These strains are particularly useful for transplantation and immunological research. Regenerative medicine, adoptive cell transfer and identification of genetically modified cells after gene therapy in vivo require the ability to track donor cells in host tissues and the EGFP rat strains facilitate these types of studies. In 2012, the RRRC received requests for EGFP rat strains from 24 academic institutions, research institutes, and for profit companies located not only in the United States but worldwide.
Example of fluorescence seen in EGFP rat strains. Pictures of organs from SDTg(GFP)2BalRrrc (RRRC:0065) animals (images on right of each panel) and wild type controls (images on left of each panel). Upper images for each panel are under bright light, bottom images for each panel are under fluorescent light. Panel A: kidney; Panel B: eye; Panel C: lung; Panel D: heart.
Future of Rodent Models in Biomedical Research
Rats and mice will continue to play a central role in biomedical research. Increasingly sophisticated manipulations of rodent models, including the creation of so called “humanized” rodents that carry human genes, cells, tissues, or organs may lead to improved and refined models for developing therapeutics for human disease. The power of comparative medicine and the use of mice and rats will continue to provide a powerful tool for advancing the understanding of both normal and disease processes across species and facilitate the transition of research from “bench to bedside” to improve human health.
Elizabeth C. Bryda, PhD, is with the Rat Resource and Research Center, Department of Veterinary Pathobiology, University of Missouri.
1. Sloane B. The Black Death in London. The History Press; Gloucestershire, UK: 2011. [Google Scholar]
2. CDC. Notes from the field: hantavirus pulmonary syndrome in visitors to a national park – yosemite valley, california, 2012. MMWR Morb Mortal Wkly Rep. 2012;61:952. [PubMed] [Google Scholar]
3. Gibbs RA, et al. Genome sequence of the Brown Nor way rat yields insights into mammalian evolution. Nature. 2004;428:493–521. [PubMed] [Google Scholar]
4. Lander ES, et al. Initial sequencing and analysis of the human genome. Nature. 2001;409:860–921. [PubMed] [Google Scholar]
5. Venter JC, et al. The sequence of the human genome. Science. 2001;291:1304–51. [PubMed] [Google Scholar]
6. Waterston RH, et al. Initial sequencing and comparative analysis of the mouse genome. Nature. 2002;420:520–62. [PubMed] [Google Scholar]
7. Cantani A. Genetic causes of hearing loss in children. Padiatrie und Padologie. 1989;24:321–330. [PubMed] [Google Scholar]
8. Morton NE. Genetic epidemiology of hearing impairment. Ann N Y Acad Sci. 1991;630:16–31. [PubMed] [Google Scholar]
9. Shearer AE, Hildebrand MS, Sloan CM, Smith RJ. Deafness in the genomics era. Hear Res. 2011;282:1–9. [PMC free article] [PubMed] [Google Scholar]
10. Jacob HJ. Functional genomics and rat models. Genome Res. 1999;9:1013–6. [PubMed] [Google Scholar]
11. Kathiresan S, Srivastava D. Genetics of human cardiovascular disease. Cell. 2012;148:1242–57. [PMC free article] [PubMed] [Google Scholar]
12. Cowley AW, Jr, Liang M, Roman RJ, Greene AS, Jacob HJ. Consomic rat model systems for physiological genomics. Acta Physiol Scand. 2004;181:585–92. [PubMed] [Google Scholar]
13. Kwitek-Black AE, Jacob HJ. The use of designer rats in the genetic dissection of hypertension. Curr Hypertens Rep. 2001;3:12–8. [PubMed] [Google Scholar]
14. Lubs HA, Stevenson RE, Schwartz CE. Fragile X and X-linked intellectual disability: four decades of discover y. Am J Hum Genet. 2012;90:579–90. [PMC free article] [PubMed] [Google Scholar]
15. Sutcliffe JS, et al. DNA methylation represses FMR-1 transcription in fragile X syndrome. Hum Mol Genet. 1992;1:397–400. [PubMed] [Google Scholar]
16. Boyle L, Kaufmann WE. The behavioral phenotype of FMR1 mutations. Am J Med Genet C Semin Med Genet. 2010;154C:469–76. [PubMed] [Google Scholar]
17. Spencer CM, Alekseyenko O, Serysheva E, Yuva-Paylor LA, Paylor R. Altered anxiety-related and social behaviors in the Fmr1 knockout mouse model of fragile X syndrome. Genes Brain Behav. 2005;4:420–30. [PubMed] [Google Scholar]
18. Colvin M, Maravelias CT. A stochastic programming approach for clinical trial planning in new drug development. Computers and Chemical Engineering. 2008;32:2626–2642. [Google Scholar]
19. Zambrowicz BP, Sands AT. Knockouts model the 100 best-selling drugs–will they model the next 100? Nat Rev Drug Discov. 2003;2:38–51. [PubMed] [Google Scholar]
Articles from Missouri Medicine are provided here courtesy of Missouri State Medical Association
Why use the mouse in research?
Humans and mice share many common genetic features and by examining the physiology, anatomy and metabolism of a mouse, scientists can gain a valuable insight into how humans function.
Key facts
An adult black mouse.
Image credit: Wellcome Library, London
Benefits of the mouse
What’s the main reason for your rating?Send
Which of these best describes your occupation?StudentScientist / ResearcherTeacherOther
What is the first part of your school’s postcode?Send
How has the site influenced you (or others)?Send
Thankyou, we value your feedback!